Going geothermal
Anyone who has peered over the edge into the caldera of an active volcano will know that there is a lot of latent power there. Every so often, we get a glimpse of it. When Mount Tambora in Indonesia erupted in 1815, it was some ten times more powerful than the more famous 1883 Mt. Krakataou (Krakatoa)[1] and blew out a cloud of sulfate so massive it engulfed the Earth. It cooled temperatures and disrupted weather patterns to such as extent there was widespread crop failure and famine was rife[2].
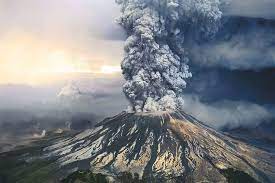
More recently, when Mount St. Helens in the US erupted in 1980, it released 24 megatons of thermal energy, equivalent to 1,600 times the size of the atomic bomb dropped on Hiroshima[3] in 1945.
Geothermal energy, derived from the heat stored within the Earth itself, offers a unique opportunity to tap into the immense energy reservoirs that our planet holds. The US Department of Energy (DOE) estimates the US alone possesses an astonishing five terawatts of heat resources – sufficient to meet the entire world’s energy needs[4].
By contrast with other renewable energy sources, geothermal energy offers both electricity and heat, as well as the potential for value-added mineral extraction. As an electricity source, geothermal energy provides reliable generation with high plant efficiency, low greenhouse gas emissions, and a small ecological footprint. When properly managed, it becomes a long-lasting and sustainable energy source that is scalable and has low operating costs.
By directly supplying heat, it increases efficiency and reduces electricity consumption for heating and cooling purposes.
Despite these inherent advantages, the development of geothermal energy faces challenges that have impeded its progress, even in regions with easily accessible resources.
Geothermal projects often encounter longer project development timelines, requiring substantial upfront capital expenditures and facing high risk during the initial exploration phases[5]. Additional hurdles stem from financing issues, policy and regulatory frameworks, institutional and technical expertise, and the need for technological advancements. These challenges affect both electricity generation and heating applications, hindering the realization of geothermal energy’s full potential to date.
Yet the situation is changing rapidly, thanks to both innovative technology and more imaginative funding programs.
Take Japan as an example[6]. After the 2011 nuclear incident at Fukushima, the Government initiated renewed support for renewable energy through a program of subsidies and feed-in tariffs (FiTs). By offering preferential prices and long-term contracts to project developers, FiTs incentivize the supply of electricity from geothermal sources to the national grid. This has sparked the construction of more than 60 geothermal plants, each generating up to 2 Mwe (megawatts electric), across 45 geothermal fields. What sets these small geothermal electric plants apart – and which could make them a model for projects elsewhere – is their lower risk profile, reduced investment requirements, and the ability to begin operation without the need for extensive exploration.
How geothermal energy works
Geothermal energy harnesses the heat stored within the Earth’s crust. It is obtained by drilling into the ground and extracting the heat using fluids, which are then brought to the surface. At the surface, the energy is extracted from the fluid and converted to electricity or used directly as heat.
Geothermal energy can be found at various depths and temperatures, with hydrothermal systems being the most widely developed. These systems use water as the conduit for the heat circulating in deep-seated permeable rocks.
The different utilization possibilities for geothermal energy depend on its temperature, which is categorized in three bands: High (above 150°C), medium (90-150°C), and low (below 90°C).
High-temperature resources are ideal for electricity production, while medium-temperature resources are used for space heating, industrial processes, and agri-food applications. Low-temperature resources are used for direct heating purposes in buildings and thermal applications such as spas and swimming pools[7].
Geothermal electricity
The primary power plant technologies used in converting geothermal energy to electricity are dry steam, flash steam, and the binary cycle[8]. Dry steam plants extract steam directly from the geothermal reservoir, which is then directed to a steam turbine coupled with a generator to produce electricity. The exhaust steam is discharged into a condenser to optimize efficiency. In smaller units, backpressure plants release the exhaust steam directly into the atmosphere, providing a simpler and more cost-effective solution.
Flash steam technology, utilizing two-phase geothermal fluids, is the most common method used in existing geothermal plants worldwide. This process involves vaporizing the geothermal fluid at a lower pressure through flashing, separating the steam component from the liquid component. The steam is expanded through a turbine coupled to a generator to produce electricity. The steam exhaust is discharged into a condenser or released into the atmosphere. Extra electricity can be generated by further flashing the separated liquid component.
Binary cycle plants transfer heat from the geothermal fluid to a secondary working fluid with a lower boiling point, generating electricity through a closed loop system. Binary plants can operate at lower temperatures and have the advantage of being able to generate electricity from geothermal resources as low as 70°-80°C.
Wellhead generators, modular units producing less than 10 MWe, offer an attractive solution for early revenue generation during the development phase of a geothermal field. They make use of existing wells, reducing the time to return on investment, and have advantages such as shorter pipelines and shorter installation times periods than larger-scale power plants.
Potential game-changer for emerging markets
More than 80 countries, including several emerging markets, are already significant geothermal power producers. In 2022, Indonesia ranked as the world’s second-largest producer with 2,356 MW, followed by the Philippines (1,935 MW), Türkiye (1,682 MW), Mexico (963 MW), and Kenya (944 MW)[9]. Some of these countries are now restructuring their financial frameworks to attract further investment and drive the pace of expansion of geothermal energy.
These emerging economies recognize the potential of geothermal energy and are adopting ambitious targets to increase its share in their energy mix. Indonesia, heavily reliant on coal for electricity generation, aims to generate 23% of its power from renewable sources by 2025. To support this goal, the Government has allocated a substantial capital expenditure budget to Pertamina Geothermal Energy (PGE), a state-owned geothermal developer. PGE is set to receive US$ 250 million in 2023, with funding expected to reach US$ 1.6 billion by 2027. This investment will enable PGE to expand its geothermal capacity from approximately 700 MW to 1,300 MW[10].
To attract investments, Indonesia has been actively seeking partnerships with foreign companies. Mitsubishi Power, for instance, has partnered with PGE to construct a 55-MW unit at the Lumut Balai Unit 2 station, with support from Japan’s International Cooperation Agency.
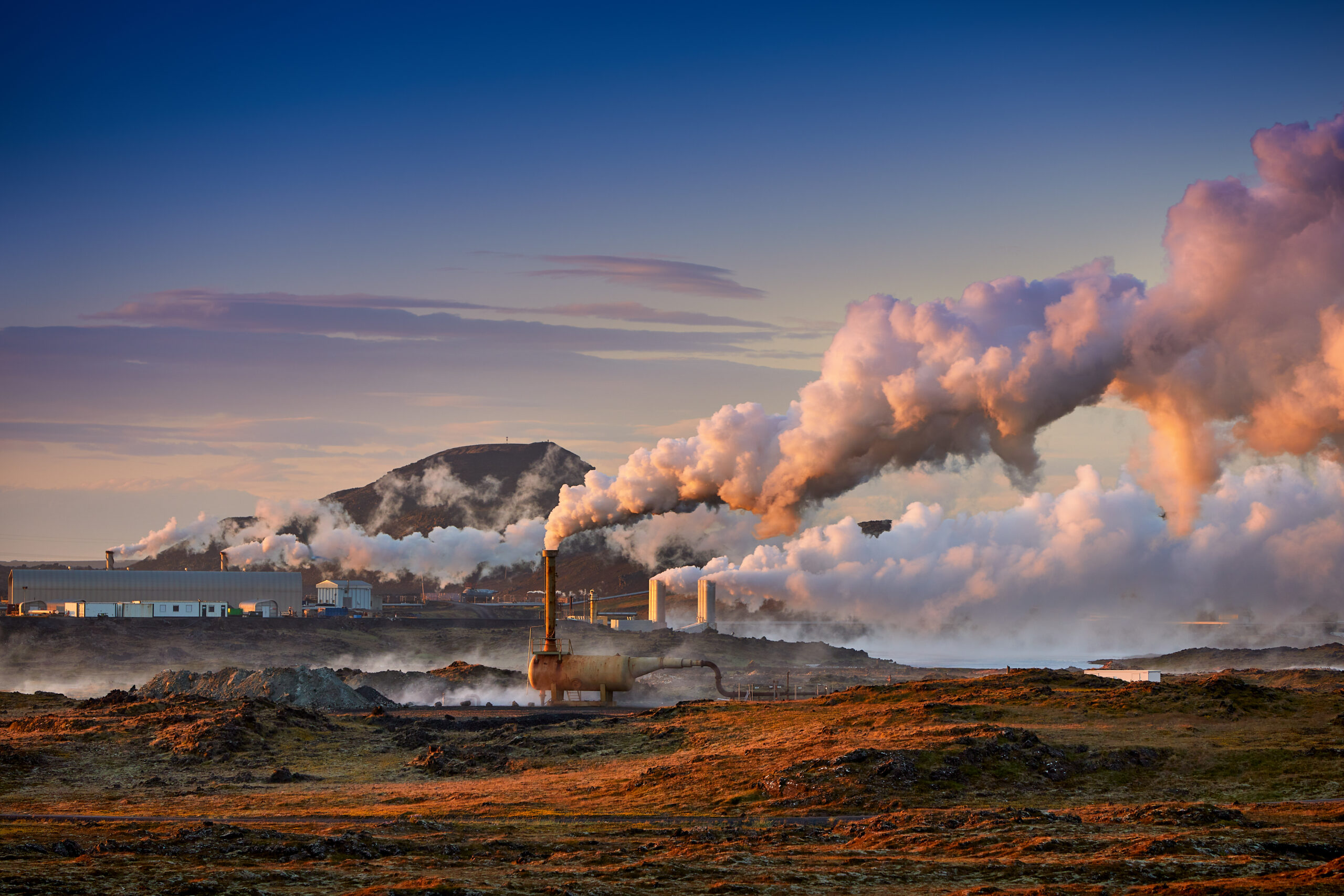
PGE and Italy’s Exergy have also signed a memorandum of understanding to study the joint development of geothermal in the country[11]. Italy is the world’s eighth-largest geothermal producer.
Indonesia is also fostering partnerships with UAE companies, such as Masdar, which made its first geothermal investment by acquiring shares in PGE’s initial public offering.
The Philippines is also committed to leveraging geothermal energy as it strives to achieve its target of renewables accounting for 50% of its energy mix by 2040[12]. The country plans to increase its geothermal capacity by 75% during this period and is currently formulating policies to drive the sector’s development. For example, Philippine Geothermal Production Company, owned by local conglomerate SM Investments, has announced plans to develop five new geothermal projects, adding 250-400 MW of capacity. To attract foreign investment, the Government has lifted the requirement for local ownership, allowing foreign companies to hold 100% ownership of such assets.
Kenya has not only built Africa’s first geothermal power plant but also, during 2020-2021, generated 48% of its electricity from geothermal sources. And it means to continue. The nation has set ambitious green development goals and aims to expand its existing 944 MW geothermal capacity to 1,600 MW by 2030, and to 10,000 MW of geothermal by 2037 – 10 times its current capacity[13].
Like Indonesia, the country wants foreign investment and know-how. It hosted the Italy-Kenya Geothermal Business and Investment Forum in March 2023 and has signed agreements with Australian renewable energy company Fortescue Future Industries and independent power producer Globeleq to invest in green energy and manufacturing. These plans include a 300-MW green energy and fertilizer facility in Naivasha that will be powered by geothermal sources[14].
Solving the finance challenge
These initiatives are immensely encouraging. One of the key factors that has shackled the development of geothermal energy to date is the cost. If you don’t have the funding yourself, you have to create the financial structures to attract and secure investors who are in it for the long-term. Geothermal projects have to become an asset class that can reasonably sit within the portfolio of a conventional investment institution, such as a pension fund.
This is recognized by the International Renewable Energy Agency (IRENA), among others, which has created a platform called the Global Geothermal Alliance to kick start knowledge-sharing and coordination among geothermal producers around the globe.
Its most recent report, Geothermal market and technology assessment[15], notes that geothermal projects require significant upfront capital and involve inherent risks, leading to a distinctive investment profile. To secure financing during the development stage, it is customary to establish long-term power purchase agreements (PPAs) ranging from 15 to 25 years.
The types of measures that can be employed at Government level to improve this situation include tax breaks and exemptions on capital expenditure for equipment, as well as operating expenses, to make geothermal energy more competitive with other sources and direct subsidies. As Japan has shown, feed-in tariffs (FiTs) are another way to bridge the cost gap between geothermal and other energy sources by offering preferential prices and long-term contracts to geothermal project developers.
Risk management is another key factor, particularly since there have been rare instances when geothermal developments have triggered earthquakes. In 2017, for example, one in South Korea catalyzed a magnitude 5.5 earthquake[16]. There is therefore a need for risk mitigation, guarantee, and insurance schemes that address uncertainties and risks associated with geothermal projects, including sub-surface risks during exploration drilling and declining well productivity. The contribution that geothermal projects make to lowering carbon emissions can also be a source of revenue through the carbon market by selling carbon credits.
Technological advances
One of the limitations of geothermal power is that it is confined to volcanic regions or areas near tectonic plates, such as the so-called ‘Ring of Fire’[17] that encircles the Pacific Ocean, as well as in the Mediterranean and East African fault lines, where existing cracks in the Earth’s core allow steam to form close to the surface.
Similar considerations have so-far seen limited development of one of the most potent sources of geothermal energy – enhanced geothermal systems (EGS). EGS utilizes geothermal resources from deeper reservoirs that are usually not economically viable due to low permeability of the surrounding rock. In EGS, fluids are injected into the ground at high speeds to fracture deep rock (known as ‘fracking’), creating pathways for water to reach accessible depths and deposits, overcoming the permeability limitations. The plus side is that EGS means that geothermal energy can produce power anywhere there is heat in the subsurface. But it is costly
All this might be about to change with the drilling technology developed by Quaise Energy[18], a US geothermal start-up. By repurposing existing gyrotron technology, typically used in nuclear fusion research, Quaise Energy’s system enables drilling up to 12 miles beneath the surface, tapping into superdeep geothermal energy sources with temperatures exceeding 400°C (752°F). This breakthrough technology eliminates the need for fracking, thereby avoiding the potential for earthquakes associated with other geothermal systems. Moreover, Quaise’s drilling technique is expected to be fast and efficient, aiming to complete boreholes within 100 days using existing 1 MW gyrotrons.
Quaise Energy sets ambitious goals for the development of its drilling technology. By 2024, it plans to demonstrate the system in the field, followed by steam extraction in 2026 and commercialization by 2028. It aims to achieve a levelized cost of electricity (LCOE) between US$ 20 and US$ 40 per megawatt-hour. This would position geothermal power in direct competition with the most competitive wind and solar energy prices, making it an economically viable and environmentally friendly energy option. With the ability to drill deeper and tap into higher-temperature geothermal resources, Quaise Technology’s approach could make geothermal power accessible to countries worldwide, regardless of their tectonic activity.
Future for fossil fuel technology
Another advantage of geothermal energy is that it can ‘piggy-back’ on both the infrastructure and the subterranean exploration experience of the fossil fuel industry. For instance, Quaise Energy plans to convert retired coal-fired power plants into geothermal baseload centers, taking advantage of their capacity and grid connections. Repurposing retired hydrocarbons fields is another avenue for generating geothermal energy. India’s Cairn Oil & Gas, for example, signed a deal with Baker Hughes to produce geothermal energy from its fields in Rajasthan[19].
Better than batteries
Research shows that EGS technology has the potential to store excess energy from solar and wind sources more effectively than lithium-ion batteries[20]. A collaboration between Princeton University and advanced geothermal developer, Fervo Energy, for example, has demonstrated that advanced geothermal reservoirs can store surplus power in the form of hot water or steam. This stored heat can later be utilized to generate electricity when renewable sources are not available. The storage capacity of geothermal reservoirs is an additional benefit without incurring extra costs during construction. This breakthrough could enable next-generation geothermal plants to depart from the traditional baseload operating model and provide significant value as providers of wind and solar energy, thereby bolstering all three renewable technologies.
Looking at the developments in both funding and technology, it’s clear that geothermal energy has a strong future. Although it faces its share of challenges, there is a growing momentum and investment in its development, indicating that progress is being made.
As of 2021, global geothermal power generation reached 16 gigawatts (GW). This may seem modest compared to the impressive capacity of the world’s largest hydroelectric plant, the Three Gorges Dam, which can produce up to 22.5 GW, yet I am convinced that geothermal energy has immense untapped power which is only just being realized.
With continued efforts and investment, geothermal energy has the opportunity to expand its footprint and contribute significantly to the global energy mix. Notwithstanding the challenges that still need to be resolved, the steady growth of projects worldwide demonstrates the increasing recognition of geothermal energy’s benefits as a reliable and renewable energy source. By harnessing the Earth’s natural heat, geothermal energy can play a vital role in achieving a sustainable and low-carbon future for our planet.
[1] The Tambora eruption although ten times more powerful than that of Krakatau, (900 miles away), is less widely known, partly because news travelled no faster than a sailing ship, limiting its notoriety. Krakatau erupted in 1883, after the invention of the telegraph, which spread the news quickly.
[2] https://experts.illinois.edu/en/publications/itamborai-ithe-eruption-that-changed-the-worldi#:~:text=When%20Indonesia’s%20Mount%20Tambora%20erupted,for%20more%20than%20three%20years
[3] https://pubs.usgs.gov/fs/2000/fs036-00/
[4] https://www.energy.gov/articles/doe-launches-new-energy-earthshot-slash-cost-geothermal-power
[5] https://www.irena.org/Publications/2023/Feb/Global-geothermal-market-and-technology-assessment
[6] https://www.irena.org/Publications/2023/Feb/Global-geothermal-market-and-technology-assessment, page 32
[7] https://www.irena.org/Publications/2023/Feb/Global-geothermal-market-and-technology-assessment, page 14
[8] https://www.irena.org/Publications/2023/Feb/Global-geothermal-market-and-technology-assessment, page 16
[9] https://oxfordbusinessgroup.com/articles-interviews/how-tech-can-unlock-geothermal-energy-in-emerging-markets/
[10] https://oxfordbusinessgroup.com/articles-interviews/how-tech-can-unlock-geothermal-energy-in-emerging-markets/
[11] https://oxfordbusinessgroup.com/articles-interviews/how-tech-can-unlock-geothermal-energy-in-emerging-markets/
[12] https://oxfordbusinessgroup.com/articles-interviews/how-tech-can-unlock-geothermal-energy-in-emerging-markets/
[13] https://oxfordbusinessgroup.com/articles-interviews/how-tech-can-unlock-geothermal-energy-in-emerging-markets/
[14] https://oxfordbusinessgroup.com/articles-interviews/how-tech-can-unlock-geothermal-energy-in-emerging-markets/
[15] https://www.irena.org/Publications/2023/Feb/Global-geothermal-market-and-technology-assessment
[16] https://www.rff.org/publications/explainers/geothermal-energy-101/
[17] https://education.nationalgeographic.org/resource/plate-tectonics-ring-fire/
[18] https://www.energymonitor.ai/tech/geothermal-can-provide-half-the-worlds-energy-quaise-energy-ceo/
[19] https://oxfordbusinessgroup.com/articles-interviews/how-tech-can-unlock-geothermal-energy-in-emerging-markets/
[20] https://www.weforum.org/agenda/2022/11/geothermal-renewable-energy-storage/