Storing a sustainable energy solution
The clean energy transformation appears is well underway. With limitless resources of solar and wind power at our disposal, global renewable energy capacity hit 3,372 GW by the end of 2022 – a year in which a record 83% of all new added capacity was renewable.[1] Yet, these numbers mask a stubbornly persistent challenge: How to contain, distribute and deploy that clean power once generated.
Modern society requires power 24/7, to heat our homes, to run our factories and to charge our technology. But rays of sunlight, like the air currents swirling through the atmosphere, do not operate to human schedules. What happens when the sun is not shining, or the blades of turbines hang dormant in windless skies?
The best, indeed, only, answer is to find a way to store this green energy, which today takes the form of batteries.
Without adequate battery technology, society will never fully free itself from fossil fuels. Instead, it will continue to rely on ‘peak power’ oil and coal generators to cover lulls in supply or to meet spikes in demand. Batteries are the ‘missing link’ in the chain between green energy supply and inconsistent, multifaceted electricity demands. They are the bridge that can help guide us to a net-zero future and potentially stave off the worst effects of climate change.
They could also give energy bills an overdue jolt. In the UK alone, a sophisticated battery storage network encompassing low-carbon power, heat and transport systems could save up to GBP 40 billion by 2050 – good news not only for environmentalists, but for struggling families, too.[2]
Lithium leading the charge to a cleaner future
The battery technologies used for storing significant amounts of green energy are typically based on lithium-ion batteries. For devices small (cellphones), medium (automobiles) or extremely large (electricity grids), lithium-ion units are generally considered the current default medium.
It is at grid-scale, however, where they have the most potential to make a sizable dent in the carbon footprint of the energy sector – a sector which currently accounts for more than 40% of all global CO2 emissions.[3]
Both economic and durable, lithium-ion batteries can be used and recharged thousands of times, can be manufactured to almost any capacity, and represent a relatively safe and low-cost solution for almost any scenario. Their superiority is evidenced by sheer dominance; lithium-ion batteries account for 95% of grid-scale systems in the market at present.[4]
Although the fundamental lithium-ion technology is now half a century old, modern iterations use algorithm-driven software to coordinate an optimum pattern of storing and releasing energy into the grid.
At a technical level, they charge and discharge power by transferring lithium ions between electrodes. Lithiated metal oxides are commonly used as the cathode for storage, and carbon as the anode for extraction.
Precise designs and chemistries vary from model to model, but lithium-ions are widely considered to have superior energy density and cost-effectiveness to any other energy storage device. Capacities can range from several KW with a few minutes of storage, to multi-megawatt variants suitable for utility substations.
Lithium-ions are not, however, perfect. Discharge rates and even climatic conditions have an impact on efficiency levels. Degradation is ultimately inevitable, of course, and the large component count of lithium-ions demand a more stringent battery management system than zinc-hybrid equivalents.
Yet confidence in the technology remains high and investment potential considerable. With momentum growing, it is estimated that some 120 to 150 new battery factories will be needed by the decade’s end to satisfy industry’s appetite for green storage solutions.[5] One study from last year suggests that the lithium-ion battery chain (covering the full journey from mineral mining to recycling) will balloon by 30% between now and 2030, achieving a market size of 4.7 TWh and a financial value of US$ 400+ million.
Even if lithium-ion units remain the runaway industry leader, research is under way on alternatives which could further turbocharge the energy storage sector.
Behold a battery of game-changing technologies
Research on substitute battery technologies – concepts that could equal or even exceed the efficiency of lithium-ions – has produced some tantalizing results.
- Sodium-ion batteries: If supplies of lithium dwindle or if the market needs to diversify, sodium-ion presents a potent alternative. Sodium-ion units have lower energy densities than lithium equivalents (120-160 watt-hours per kg versus 170-190 watt-hours per kg) and shorter life cycles (2,000 to 4,000 recharges versus 4,000 to 8,000) but are up to one-fifth cheaper to produce.[6] Being less prone to ‘thermal runaway’ (uncontrollable heating of flammable electrolytes) they may also be considered as potentially safer. In 2023 alone, at least six new manufacturers started production of sodium-ion batteries.
- Flow batteries: These giant rechargeable fuel cells produce energy via a pair of chemical components dissolved in liquid and divided by a membrane. When operational, electrolytes are pumped from tanks through electrodes to extract the electrons, before being recharged and returned to the storage tank. Early flow batteries required vanadium, a rare and costly metal, but some later iterations swap vanadium for more sustainable organic compounds that also grab and release electrons. Such devices could one day power thousands of homes for many hours. While flow batteries have lower energy densities and discharge rates than many solid electroactive materials, they are rapidly proving themselves viable at utility scale. The Chinese port city of Dalian last year began operating a 400 MWh, 100 MW flow battery, allowing inhabitants to draw extensively on wind and solar power.
- Compressed air energy storage: In this system, surplus energy is used to compress air and store it within a large, pressurized container, before gradually releasing it through a revolving turbine to generate electricity. To minimize costs, compressed air can sometimes be stored in existing features such as disused salt mines. While the technology is not new, research is currently under way on methods to store the heat generated during compression for reuse as an expansion catalyst when the time comes to discharge power. In the UK, a university-led project is investigating the use of deep-sea ‘energy bags’ as storage vessels. Using this method, the cost per unit of energy stored could fall to GBP 1-10 / kWh, considerably cheaper than pumped storage (GBP 50 / kWh) or electrochemical storage (GBP 500 / kWh).[7]
- Mechanical gravity batteries: these systems use weighted blocks that are winched up a tower, then allowed to descend during periods of energy deficit to generate electricity via natural gravitational force. It makes sense – what goes up must, after all, come down. The mass of a gravity battery combined with its gradual descent creates a vast amount of torque, generating maximum power instantaneously. Such mechanical gravity batteries can also help maintain grid equilibrium, eradicating the kind of fluctuations that risk blackouts and infrastructure damage. Swiss-based Energy Vault is this year putting the finishing touches to two gravity battery projects, each designed to showcase the technology’s versatility. One, near Shanghai, China, will have an energy storage capacity of 100 MWh to power 3,400 homes. Another system, in Texas, USA, features a 460-foot-tall structure with a total energy capacity of 36 MWh.[8]
- Sand batteries: These in-development devices use sand (or similar substitute) as a high temperature heat storage medium, typically for energy generated from wind or solar sources. Once deployed, the energy can be used to warm buildings directly, or to provide steam for industrial processes typically reliant on fossil-fuels. Although the technology awaits mass adoption, an early working example already exists in Finland. Operated by utility company Vatajankoski, the sand battery in the town of Kankaanpää heats a range of commercial and domestic buildings. The kit, comprising an insulated steel silo filled with sand and heat transfer pipes, can reach around 600 degrees Celsius and hold up to 8 MWh of thermal energy when full. With its high temperature tolerance, sand can hold several times the amount of energy that could be contained in an equivalent water tank – although converting that heat back into electricity currently has an efficiency rate of just 30%. Sand batteries could prove a durable solution, the absence of any chemical reaction meaning they are spared the wear-and-tear process of regular batteries. The team behind the Finnish project has high hopes for the technology, believing sand batteries could one day be viable for any location around the world with a district heating system.[9]
To maximize the potential of battery storage technologies, stakeholders must funnel funds towards the right areas of research and ensure large-scale industrialization. To benefit both the environment and the bottom line, the sector must focus on sustainability, transparency, and resilience along the entire value chain.
Short-circuiting the problems of battery adoption
Certain challenges – all vexing, but none insurmountable – are expected along the journey to a battery-powered future.
Mining and refining rare earth minerals depletes natural resources, degrades land, creates waste, contaminates soil and water, and leads to biodiversity loss. In emerging markets, where many of our critical battery components are sourced, problems can arise over issues such as indigenous rights and forced labor.
Lithium carbonate demand is expected to exceed 3,000 kilotons by 2030, far surpassing the circa 600 kilotons produced in 2021 and the circa1,500 kilotons forecast by 2030, under current mining deals.[10]
Stronger regulations, increased transparency and more farsighted planning can help public and private sector stakeholders navigate potential ESG (environmental, social and governance) pitfalls.
Historically, the battery value chain has also been hampered by price volatility. In a global cost of living crisis, decarbonization could be fatally delayed by high battery costs. Against this backdrop, it remains unclear how the industry could sufficiently scale-up to meet future needs.
There are also the shifting sands of national policies to consider. Greater cohesion is needed to withstand the challenges of disrupted supply chains, a shortfall in skilled labor, and disunity on international intellectual property protection.
Mitigation strategies can help, if promptly introduced. Long-term contracts and vertical supply chain integration could help provide certainty and boost investor confidence. To win vital hearts and minds, the disruptive mining of rare earth minerals must be tied into a wider social movement and growth agenda.
With batteries repeatedly demonstrating their environmental value across the whole production-to-disposal timeline, the industry has no intention of standing still. But what will success look like? According to the experts, a healthy, sustainable battery ecosystem by 2030 will have three mandatory characteristics:[11]
- A resilient supply network: In an ideal scenario, regions would largely source their own materials to satisfy domestic demand.
- Sustainability: To decarbonize our society, energy storage industries must actively decarbonize themselves, ensuring a 90% drop in material and manufacturing emissions by the end of the decade.
- A circular value chain: Materials approaching the end of their life must be rigorously reprocessed and fed back into the system. Such a culture will minimize waste while extracting maximum economic value, thought to represent an additional US$ 6 billion in profit by 2040.
Harmonizing the energy storage ecosystem will require enthusiastic support from lawmakers embellished by bold vision from within the private sector. Disruptors and innovators like Abdul Lateef Jameel can help usher in this brighter future.
Private sector brings power to the people
Fotowatio Renewable Ventures (FRV), Abdul Lateef Jameel’s flagship renewable energy business, focuses not just on generating green electricity from wind and solar, but also on pioneering energy storage projects.
In 2022, it won funding for its latest battery energy storage system (BESS), a 250 MW lithium-ion plant in Gnarwarre, Victoria, Australia. The deal came hot on the heels of its agreement to build the 100 MW Terang BESS, also located in Victoria.
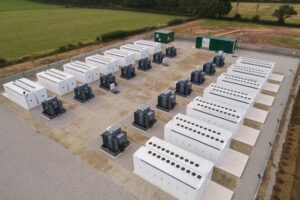
FRV’s innovation arm, FRV-X, is developing a reputation for utility-scale BESS schemes worldwide, guaranteeing round-the-clock power supplies for homes and industries.
Already FRV is involved in BESS projects in the UK at Clay Tye, Essex (99 MW / 198 MWh); Holes Bay, Dorset (7.5 MW / 15 MWh); and Contego, West Sussex (34 MW / 68 MWh).
It also operates a hybrid solar and BESS plant at Dalby, Queensland, Australia.
In 2022, FRV purchased two additional BESS projects in the UK, plus a majority stake in a BESS project in Greece. FRV-X has more than 500 MW of battery storage projects operational across a swathe of key markets.
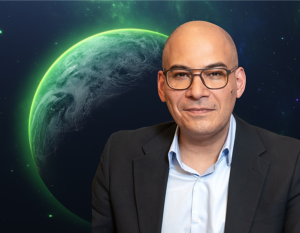
Deputy President & Vice Chairman
Abdul Latif Jameel
“We are proud to be leaders in sustainable energy storage innovation, helping to achieve a more viable future for us all,” commented Fady Jameel, Deputy President & Vice Chairman of Abdul Latif Jameel.
“From a technological perspective, we are mastering the art of generating green energy cleanly and economically. Efficient electrical storage systems are the missing link in our pathway to a truly sustainable society. There is great excitement within the industry at the current rate of evolution of battery technology, and we are proud to be a part of that concerted effort.”
The data inspires great confidence. Global battery markets could safeguard up to 18 million jobs by 2030, both by creating new positions and by securing existing ones.[12] In the road transport sector alone, the coming battery revolution could cut greenhouse gas emissions by up to 70 gigatons of CO2 equivalent (GtCO2e) by 2050.
The push for dependable, versatile green energy is not unique to one particular climate or culture. Rather, it is being aggressively bankrolled the world over. In the USA, thanks to the 2022 Inflation Reduction Act, stakeholders are vying for a slice of the US$ 370 billion kitty earmarked for clean-energy investments. Across the Atlantic, in Europe, an unfolding energy crisis triggered by the Russia-Ukraine situation is encouraging an innovation-first reappraisal of supply and storage. The EU Green Deal seeks to mobilize at least €1 trillion of environmental investments in clean energy and sustainability over the next decade.[13]
Little surprise, then, that the BESS sector is anticipated to grow at an average of 29% per year from now until 2030.
The 450 to 620 GWh of annual utility-scale installations will establish 90% market share by that year.[14] Too ambitious? Never. Securing a meaningful network of utility-scale battery storage facilities means releasing the handbrake, once and for all, on our imperative green transition.
[1] https://www.irena.org/News/pressreleases/2023/Mar/Record-9-point-6-Percentage-Growth-in-Renewables-Achieved-Despite-Energy-Crisis
[2] https://www.nationalgrid.com/stories/energy-explained/what-is-battery-storage
[3] https://documents.worldbank.org/en/publication/documents-reports/documentdetail/873091468155720710/Understanding-CO2-emissions-from-the-global-energy-sector
[4] https://www.windpowerengineering.com/how-three-battery-types-work-in-grid-scale-energy-storage-systems/
[5] https://www.mckinsey.com/industries/automotive-and-assembly/our-insights/battery-2030-resilient-sustainable-and-circular
[6] https://www.mckinsey.com/industries/automotive-and-assembly/our-insights/enabling-renewable-energy-with-battery-energy-storage-systems
[7] https://www.thegreenage.co.uk/tech/compressed-air-energy-storage/
[8] https://interestingengineering.com/innovation/two-massive-gravity-batteries-are-nearing-completion-in-the-us-and-china
[9] https://www.bbc.com/future/article/20221102-how-a-sand-battery-could-transform-clean-energy
[10] https://www.mckinsey.com/industries/automotive-and-assembly/our-insights/battery-2030-resilient-sustainable-and-circular
[11] https://www.mckinsey.com/industries/automotive-and-assembly/our-insights/battery-2030-resilient-sustainable-and-circular
[12] https://www.mckinsey.com/industries/automotive-and-assembly/our-insights/battery-2030-resilient-sustainable-and-circular
[13] https://eucalls.net/blog/the-basics-of-the-european-green-deal
[14] https://www.mckinsey.com/industries/automotive-and-assembly/our-insights/enabling-renewable-energy-with-battery-energy-storage-systems